Trends in Energy Technologies for 2023
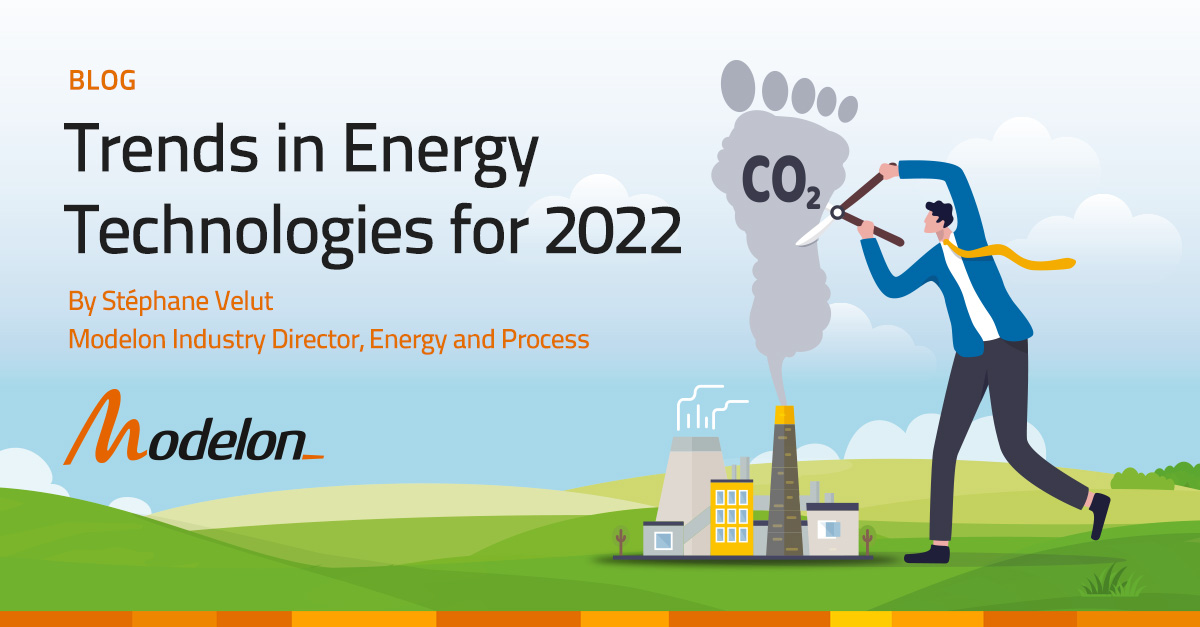
This blog describes the trends and observations of innovative energy technologies in 2023 and beyond. With over 20 years of experience in model-based engineering, Modelon’s Energy and Process Industry Director, Stéphane Velut, shares his views on energy technologies trends, observations & insights – with a focus on what he is seeing in the industry and how simulation software is enabling world-leading organizations to get ahead.
Organizations have made a goal of significantly reducing CO2 emissions to mitigate climate change. In addition, governments and energy companies are now investing in renewable energy sources, moving away from fossil fuels. Model-based design and system simulation have helped to support innovation and combat the costly inputs that many of these large-scale projects bring about. Here are a few emerging trends in energy system projects for 2023 and years to come that Modelon witnesses and supports.
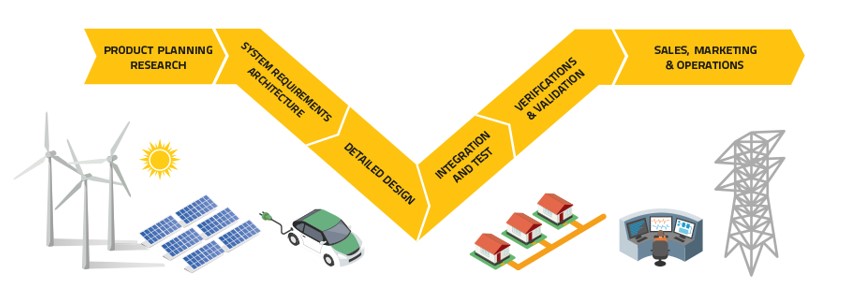
Trend 1: Energy industry focused on carbon neutrality
With large investment in mature and low-cost renewable energy technologies such as wind and photovoltaic (PV), the energy industry is leading the charge on decarbonization. To support the rapid deployment of renewables without jeopardizing power systems’ stability, dispatchable units, including fossil fueled plants, are currently balancing the production and demand of power. This will certainly be the case until large-scale energy storage systems are commonly available.
To address CO2 emissions of existing assets, energy companies have regained interest in Carbon Capture and Storage technologies. Carbon Capture and Storage, also known as CCS, is the process of seizing carbon dioxide before it enters the atmosphere, transporting it into a storage location, and isolating it for years to come. Post-combustion CO2 absorption using liquid amine solvents has been in focus for 15 years, but unfortunately, has not been commercially deployed at the expected pace due to the lack of incentives and carbon taxes. However, this is now changing. Utility companies are now requesting academic engineering departments and commercial tools to estimate the cost of CCS and enable stable CO2 removal rates while minimizing the impact on the power plant, like reduced power production or constraints on transient operation.
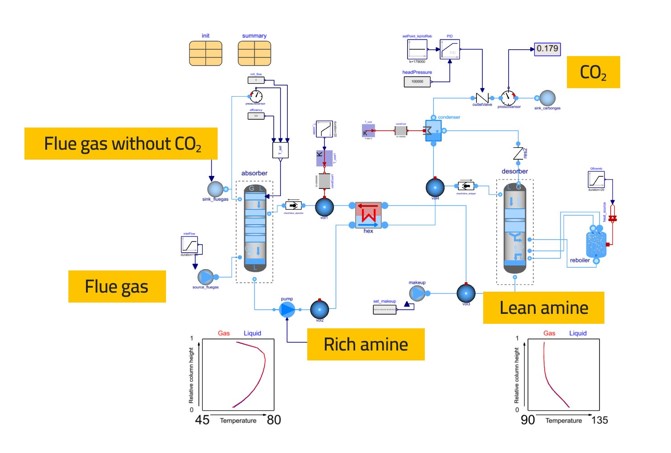
CCS can also accelerate the deployment of hydrogen technologies by making the steam reforming of methane a cheap and cleaner way of producing hydrogen until electrolysers have become more affordable.
A concept related to CCS is CCUS which means Carbon Capture, Utilization, (sometimes referred to as ‘Usage’) and Storage. CCUS follows the same idea as CCS but instead of storing carbon, it is repurposed for industrial processes by converting it into either plastic, concrete, or fuel. The production of synthetic fuel such as diesel or methane can also involve direct air capture (DAC) instead of point source capture. DAC is in the early stages, but is a path being explored by companies from all sectors. This is because it has the potential to make the transport sector carbon-neutral while leveraging the existing hydrocarbon infrastructure.
Although carbon capture has been identified as necessary technology to achieve climate targets, the main challenge is commercial deployment. This requires heavy investments in design, build, and operation costs. Many companies have turned to model-based design and system simulation to assess the available options for a cost-efficient implementation of meeting targets in CO2 emission reduction.
Trend 2: Energy technology storage systems are in the spotlight
Energy comes in multiple forms – mechanical, electrical, thermal, and chemical – so the means and technologies of converting and storing energy vary greatly, yet are plentiful.
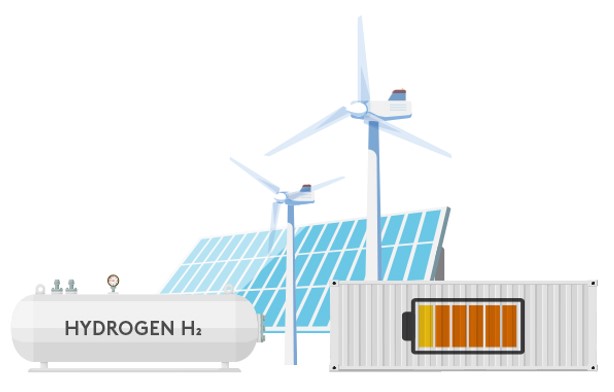
Energy storage systems have emerged as key enablers for a wide deployment of renewable energy sources. The main goal of storage projects in power applications is to store the renewable power surplus and use it later. The challenge for companies is to find a cheap, efficient, scalable, and dispatchable solution using standard components and materials for the creation of these storage systems.
As more and more companies prioritize and budget for energy storage systems, Thermal Energy Storage (TES) systems are emerging to be the frontrunner for these companies. TES is not only relevant for heating and cooling applications (power-to-heat), but also for storing and converting heat into electricity. Heat stored at high temperatures can be efficiently converted into power using standard heat engines such as the ones used in existing thermal power plants. The options investigated to cheaply store heat at a large scale include concrete, stone, sand, or molten salt. Examples of technologies where TES systems are used for power generation include:
- Pumped heat – renewable power is converted into heat using a heat pump which is then converted back to power within a heat engine.
- Concentrated solar power (CSP) – solar radiation is concentrated and stored as heat which is then converted to power using a heat engine.
- Retrofitted traditional boilers – TES is installed at an existing plant to store renewable power from the grid as heat and converted back to power using the installed steam cycle.
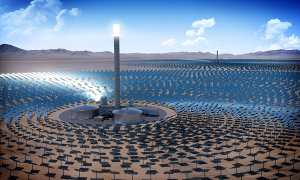
Source: https://helioscsp.com/how-to-achieve-us63mwh-in-a-concentrated-solar-power-tower-project-with-storage/
All solutions above involve the assembly of many standard components including pumps, valves, tanks, turbines, etc., that need to be designed and controlled in a smart way. System simulation is very well suited to select the optimal system configuration and derive control strategies for normal operation (start-up, shutdown, charging, and discharging) or failure modes.
Trend 3: Hydrogen fuels the future
To simultaneously address trends 1 and 2, many companies are turning to the most abundant element in the universe – hydrogen. Hydrogen is an incredibly versatile energy source with the potential to replace many existing technologies, bringing forth more sustainable and long-lasting alternatives for every industry.
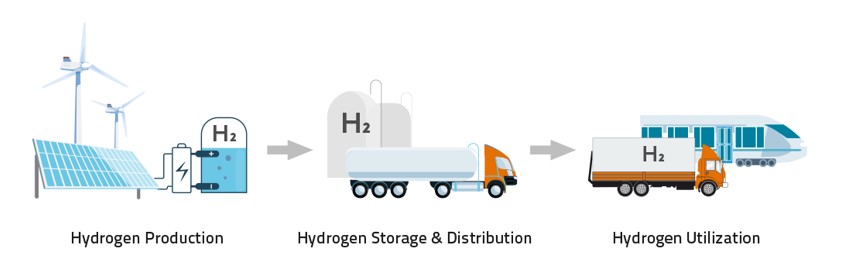
Governments are heavily investing in hydrogen related projects and there is significant research and development work ahead of us. Design and simulation tools at the component and system level can help engineers shorten the development time and make the right decisions at every stage of the value chain.
The Component Level
At the component level, there is a general need to improve design with respect to efficiency, safety, and cost. Certainly, the most challenging task is lowering the cost of green hydrogen production in electrolyzers. In the near term, producing hydrogen [as mentioned in Trend 1] by combining steam reformation of methane and CCS is clearly a good trade-off between sustainability and cost.
On the utilization side, energy companies are planning to increase the share of hydrogen as fuel in gas-fired power plants. This can be achieved as explained in our previous hydrogen power blog. Additionally, fuel cells for stationary and mobile applications aims at increased performance and lower production costs. System simulation can help to improve the overall design and control of fuel cells, from the air and fuel supply to water and heat management.
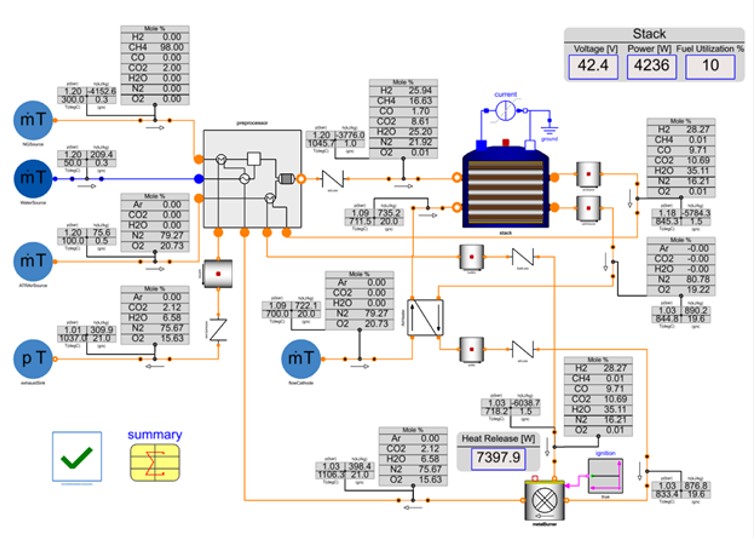
The System Level
At the system level, there is a need for tools that helps decision makers assess and minimize the cost of the transition to hydrogen technologies. The transition is certainly going to happen carefully, and various hybrid configurations and energy technologies will need to be considered. Here are examples of questions decision makers are asking that system design and simulation solve:
- Based on the current and forecasted price for electrolyzers and hydrogen, should hydrogen be locally produced or purchased?
- What is the best storage option to store energy surplus from my local renewable energy source – either hydrogen or battery?
- What is the impact of governmental incentives, renewable energy credits, or carbon taxes have on our optimal investment? Is it better to invest in CC(U)S to quickly reach carbon neutrality with my existing fossil fueled assets or to directly switch to a hydrogen-based solution?
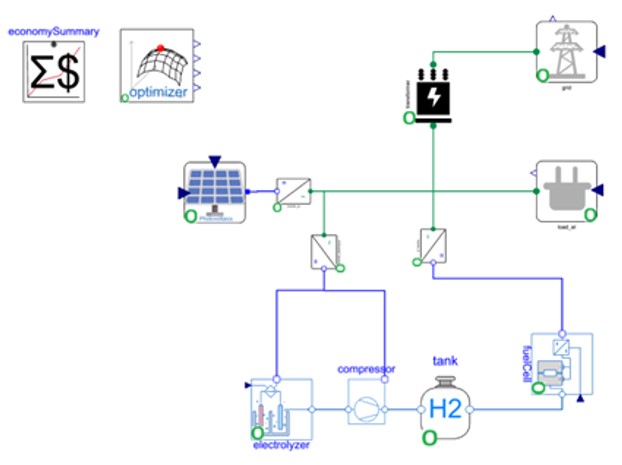
Conclusion
Energy systems innovation plays a major part in the sustainability of our planet and reducing carbon dioxide emissions. CCS, DAC, energy storage systems, and hydrogen technologies are on the rise in innovative energy solutions for 2023 and beyond. Model-based design and system simulation help innovators plan the design and optimization of these large-scale projects for a successful outcome of bettering energy use for the future. To learn more about the ways in which model-based design and system simulation can help your organization become more energy efficient, contact us today.