Cogeneration Power Plant Optimization for Managing Renewable Energy Efficiently and Economically
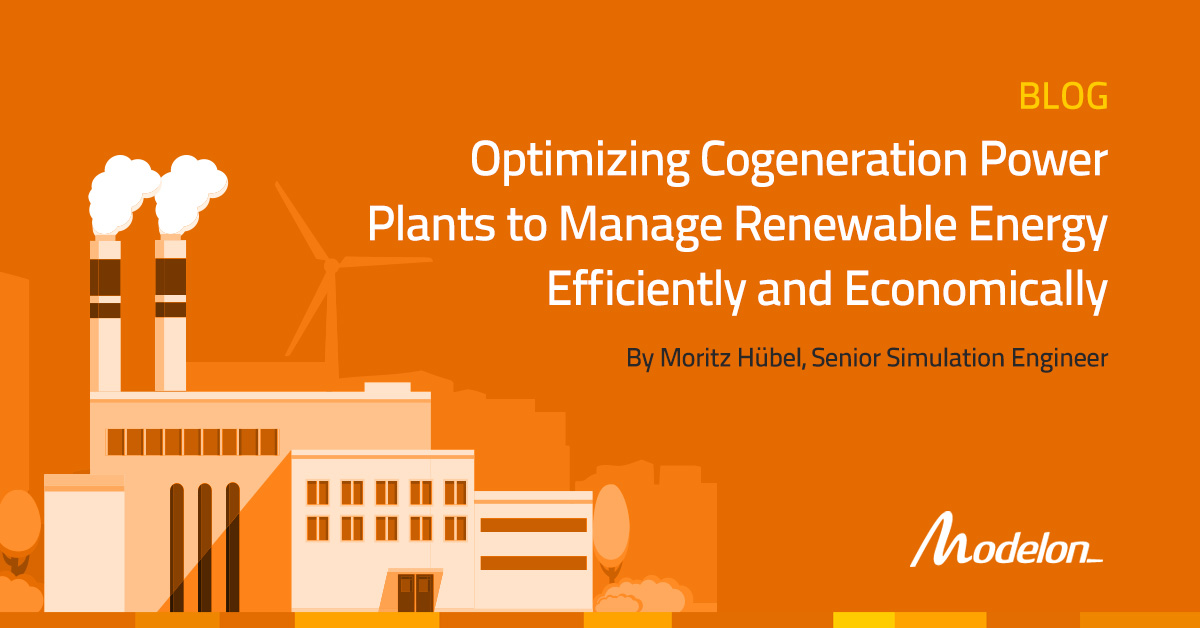
Cogeneration, or Combined Heat and Power (CHP), plant uses a heat engine or power station to produce electric and thermal energy simultaneously from a single fuel source. A primary benefit of using a cogeneration system is that it can capture thermal energy for heating that is otherwise wasted in a conventional power plant.
Is it efficient? Yes.
Is it sustainable? Not yet.
Cogeneration and the Challenge of Transitioning to Renewable Energy
Utility companies today face the challenge of transitioning to the utilization of renewable energy for both electricity production and district heating systems. Several factors contribute to the difficulty of such a transition:
- Renewable Source Instability: In energy systems with a high share of conventional generation, each unit could provide a small amount of control power. Traditional gas-fired cogeneration plants did not need to perform short–term power adaptions and provide ancillary service to stabilize the electrical power grid. However, as the share of renewable energy sources increases, cogeneration units can be used to compensate for the reduced control power to stabilize the electrical grid.
- Economic Uncertainty: With the many variables that accompany the addition of renewable energy sources to a cogeneration power plant, electricity prices become more unpredictable. Because cogeneration plants supply both heat and electricity, their energy output needs to be adapted to address these economic concerns, resulting in an increasing number of load changes. But such an increase results in higher component stress and increased maintenance costs.
- Decoupling Energy: Decoupling electricity production from heat production will become highly relevant in the future pursuit of energy efficiency. For example, utility companies will need to decouple output more efficiently in times of high electricity production from wind energy with a simultaneous increase in heat demand. To accommodate such factors, thermal energy storage systems are increasingly being used for district heating systems. However, integrating thermal storage systems into existing power plants and establishing efficient operational strategies are ambitious engineering tasks.
Martin Brauer, head of System Development at Stadtwerke Rostock AG, an energy service provider in Rostock, Germany, realizes this challenge: “For the last few decades, we have been generating electricity using our highly efficient gas-fired cogeneration plants to provide a reliable source of energy for our customers. In order to prepare our generation portfolio for the future we want to reduce emissions even further. We believe utilization of thermal heat storages and flexibilization of our generation portfolio are necessary to allow higher shares of renewable energy, especially from fluctuating sources such as wind and photovoltaics to be integrated.”
Modeling and Simulation Software Helps Optimize Load Changes
In thermal power plants, load changes introduce temperature and pressure changes. Thick-walled components are especially affected by this change because their high thermal inertia causes outer temperatures to change slowly even when rapid changes occur with the inside fluid conditions. The different temperatures cause the material to contract or expand, causing both thermal stress and increased pressure due to mechanical stress. Monitoring and reducing such stressors is crucial to enhancing the flexibility of power plants and necessary to integrate more renewable energy sources.
Modelon’s Thermal Power Library (TPL) provides a comprehensive modeling, simulation and optimization framework to address these engineering challenges. It contains off-the-shelf models for district heating systems and thermal cogeneration power plants using heat storages. The 2019.2 release of TPL also provides ancillary service examples and thermal and mechanical stress calculation models based on the EN 12952 standard for water-tube boilers and auxiliary installations.
Using simulation models from TPL, example transients for temperature and pressure can be set in the high pressure, high temperature part of a conventional power plant boiler during start–up, as presented in Figure 1. Temperature and pressure are increased to reach the nominal point at 600°C and 210 bar. As a result, disturbances of both temperature and pressure may occur. In this example, a 25 K temperature drop is given as an input.
Figure 1: Temperature and pressure transients during the startup of a thermal power plant.
The resulting dynamic wall temperature in the header as a basis of the thermal stress calculation can be simulated using the heat conduction models included in TPL. Figure 2 shows the time–dependent temperature distribution for the fluid temperature trajectories during the start–up process.
Figure 2: Radial metal temperature distribution of a thick-walled header component during a power plant start–up with temperature disturbance of the fluid temperature shown in Figure 1.
Figure 3 shows the resulting mechanical stress caused by the fluid pressure, the thermal stress caused by the wall temperature difference, and the combined total stress of both transient effects.
Figure 3: Mechanical, thermal and total stress for a thick-walled cylindrical header component.
For the maximum overall stress amplitude that determines the lifetime consumption of such a header component, the addition of thermal and mechanical stress clearly result in an optimization problem to find the right wall-thickness for a component for different transients. Such a task can now easily be automated using the latest wall stress models in TPL, as Figure 4 shows.
Figure 4: Maximum total stress amplitude of a thick-walled header during start–up with regular and doubled gradient showing the dependency on the wall thickness. For a regular start-up, the component should be designed with a wall thickness of 0.075m for minimum stress. For a faster start-up with doubled gradient, the stress is generally higher with its minimum at 0.055m wall thickness.
Besides the stress analysis based on EN 12952, the latest TPL release includes a comprehensive collection of other application example models. These models address other typical engineering challenges such as increasing ancillary services and integrating thermal energy storages.
Dynamic Power Plant Modeling Leads to Advances in Technical Optimization
Stadtwerke Rostock AG has been using a Thermal Power Library–based dynamic model of their power plants to address its technical challenges, including an investigation into the circumstances contributing to thick-walled header component stress in order to achieve optimal flexibility.
Researchers at the University of Rostock and the FVTR GmbH developed the system model of the power plant. As Hinrich Prause, the model creator, explains: “We started to develop a dynamic model to investigate the optimization potential for providing ancillary services, but we have also used it to evaluate the impact on thermally stressed components during those transients. We used it to investigate the best way to integrate thermal heat storage into the energy system as well.”
Martin Brauer, who initiated the research on the model-based investigations with the University of Rostock some years ago, recalls: “We did the best preparation for different technical projects by investigating everything beforehand and having an insight into every detail using the dynamic model of our power plant. We appreciate the opportunity of having a flexible tool that can be easily adapted to various applications. With that model we can do investigations that would otherwise require expensive and risky testing with the real plant or not be possible at all when it comes to the integration of new components.”
Stadtwerke Rostock has successfully adapted its conventional units and they are now participating in the ancillary service market. Furthermore, a large-scale thermal energy storage system is currently under construction at the power plant site. Some of case studies about using dynamic models to optimize cogeneration plant efficiency have been published in academic literature [1], [2].
References
[1] Wittenburg, R., Hübel, M., Hassel, E., Effects of rising dynamic requirements on the lifetime consumption of a combined cycle gas turbine power plant, International Conference on Applied Energy, August 22-25 2018, Hong Kong, China.
[2] Prause, H., Hübel, M., Nocke, J., Hassel, E., Dynamische Simulation von Gas- und Dampfturbinenkraftwerken mit Kraft-Wärmekopplung, 14. Symposium Energieinnovation, 10.-12.02.2016, Graz, Austria. (ISBN: 978-3-85125-447-1).