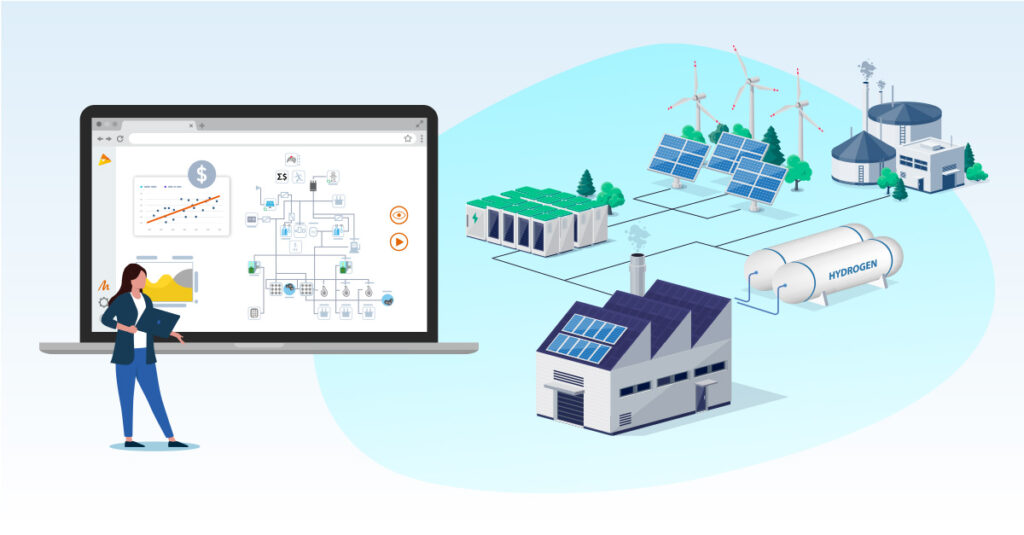
Most important industries in the United States are energy-intensive and extremely reliant on fossil fuels. Fossil fuels are cheap, reliable, available, and easy to control. But they have never been sustainable. We need to move quickly and take concrete steps to implement alternative approaches to industrial processes.
According to the U.S. Department of Energy, cutting 14 percent of emissions across eight key industrial sectors in the United States is feasible by 2030. Unfortunately—despite efforts to incentivize industrial decarbonization—at the current pace, the share of emissions from these key U.S. industries is set to rise by 2030.
Several factors delay our progress. Some of these are technological, but more often, a combination of economic, political, and even psychological factors create obstacles to decarbonization efforts. The result has been a broad “reluctance among companies to be a first mover,” according to the Department of Energy. That reluctance is largely driven by uncertainty.
Decision makers in the industrial and manufacturing sectors need to make investment decisions today for systems that last decades. Meanwhile, it’s extremely difficult to determine what mix of renewables, low-carbon solutions, and carbon capture technologies to deploy—and when to deploy them—especially when you factor in fluctuating prices and interest rates, carbon taxes, and government incentives.
Engineers, designers, and decision-makers need new, more comprehensive tools to bring these factors together in one place.
Learn how Modelon helps energy suppliers, equipment manufacturers, and start-ups use model-based design and system simulation to plan decarbonization efforts and system investments.
An Overview – Getting Decarbonization Back on Track
Eight key industrial sectors (identified by the U.S. Department of Energy) account for 14 percent of U.S. carbon emissions—roughly 880 million tons of carbon dioxide annually. These are: chemical manufacturing (by itself responsible for 7 percent of total U.S. carbon emissions); petroleum refining; iron and steel production; food and beverage processing; pulp and paper processing; cement production; aluminum production; and glass manufacturing.
These sectors aren’t just vital to our current way of life; they are also vital to a global clean energy transition, efforts to discover and improve renewable energy sources, and the broad deployment of decarbonization technologies. Any decarbonized economy must address the emissions associated with these key industries.
However, current decarbonization efforts in these industries have been limited to a small subset of relatively straightforward technological strategies. These methods don’t require large investments or process changes and focus primarily on energy efficiency, electrification, and alternative fuels (like hydrogen instead of natural gas). Unfortunately, even though this is the “low-hanging fruit,” full-scale adoption has not yet begun, and even if these measures were broadly adopted across the highest emission-producing industries, they would only yield a 10 percent reduction in carbon emissions in those eight sectors.
Broad progress toward deep decarbonization in the U.S. needs to catch up with both international efforts and our own domestic net-zero targets; most industries limit current short-term solutions to strategies with reliably predictable costs and benefits. We need industry-wide decarbonization efforts that go deeper and move faster.
Cloud-based, multi-fidelity, physics-based modeling tools can help diverse teams easily collaborate on various design and control approaches. With these tools, they can forecast the effect of different environmental, technical, and economic conditions on their industrial processes and then use that knowledge to find the best balance of performance and cost over the broadest range of conditions.
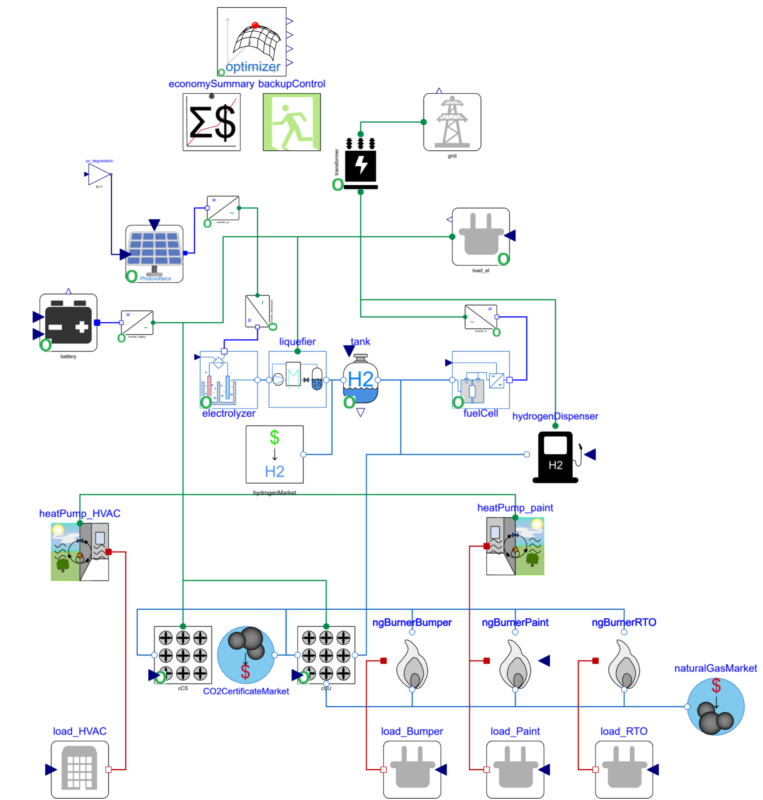
The Business Case – Techno-Economic Modeling for Industrial Decarbonization Problems
Broad industrial decarbonization is uniquely challenging to implement because it incorporates a wide range of technological, economic, and political factors. However, the right design and simulation tools can decrease development time and support best practices at every stage of the value chain—not just at the component level, but at the system level. And such tools can do so in a single unified model. The right simulation tool gives designers and decision makers the visibility they need to assess their technological options against various KPIs.
For example, at the system level, comprehensive, multi-fidelity, physics-based dynamic models are already helping organizations make the most of their transition to hydrogen energy technologies. Manufacturers and energy producers in many industries are using multi-fidelity models to help them weigh the effects of governmental incentives, renewable energy credits, and carbon taxes on their plans to either invest in Carbon Capture and Storage (CCS) technologies to reduce their carbon footprint or switch immediately to hydrogen-based or other renewable solutions.
By adopting more comprehensive, multi-fidelity, techno-economic models, engineers and decision-makers can account for the effect of economic and political realities on complex technical systems, better prepare for different future scenarios, and confidently make long-term investment decisions today.
The Solution – Specialized Modeling Tools for Technological and Economic Optimization
Modelon Impact is a cloud platform for designing, simulating, and analyzing physical systems using model-based design. Through its Thermal Power and Energy Systems libraries, Modelon Impact can create comprehensive, flexible models that meet the needs of a range of industrial sites—from individual manufacturing plants or single-building microgrid solutions to municipal-scale district heating systems. Whereas most modeling solutions allow for either a fixed control structure (within which you may explore component sizing) or fixed component design (which allows control strategy analyses), Modelon’s newest library can do both simultaneously.
The new Energy Systems Library (ESL) features a Techno-Economic Optimization Framework, which is a one-of-a-kind tool, offering users an actual mathematical design and operation optimization of their facilities or systems. With the Techno-Economic Optimization Framework, users can simultaneously explore the optimum size and control strategy at the same time and evaluate those at different fidelities, prices, interest rates, emission taxes, and economic conditions, with capital expenditures (CAPEX) and fixed or variable operating expenditures (OPEX) assigned on a per component basis (as needed).
ESL allows for technological and strategic assessment across various KPIs, including CAPEX, OPEX, Total Cost of Ownership, Net Present Value, Payback Time, and more. This library is based on the previous Energy & Process Microgrid package and is optimization-ready for decarbonizing industries.
Energy Systems Library
The Use Case – Industrial Site Decarbonization Using Modelon Impact
Industrial manufacturers are already using Modelon Impact to take on the challenge of decarbonizing their U.S. operations. For example, Modelon has worked closely with Honda, which has pledged to be entirely carbon-free by 2050. The first step in this endeavor is a pilot program to plan the decarbonization of a U.S.-based assembly plant. For this pilot, Honda wanted to take a broad view to determine which investment to make (and how to time those investments) to get the most significant carbon emissions reductions at the most sustainable cost.
To make these design decisions efficiently, the team needed a model that accurately represented an existing U.S. assembly plant, factoring in diesel, electrical, propane vehicles; photovoltaics; hydrogen; batteries; and fuel cells. The model also had to factor in economic and practical needs like surface area for photovoltaics, minimum energy storage required for backups, seasonal energy demand paired with regulatory caps, peak shaving strategies, etc.
Modelon ultimately built Honda’s model on the Impact platform, relying on the Techno-Economic Optimization Framework built into the Energy Systems Library. The model included varying electric, hydrogen, and thermal loads for buildings, transportation, and HVAC systems. Rather than design a control strategy for each plant configuration under consideration, the client used Modelon Impact’s native solver to optimize for both component sizing and control strategy simultaneously.
Ultimately, this helped them determine the total cost of operation (based on client estimates for fixed costs and operational costs for each component) while accounting for costs associated with peak shaving and the demand charge for high-demand seasons, laying the groundwork for their decisions moving forward.
Related Resources
Customer success is always our top priority. During the past two decades, we’ve distinguished ourselves by delivering exceptional service and expertise, earning a reputation as a trusted partner in many industries.
We know that a complex new tool can be daunting; that’s why Modelon regularly releases easy-to-follow tutorials and guides in the Modelon Help Center. Need further support? Experts are here to help you and your team move forward.
Ready to make an impact with system simulation?
Get in touch today to see how Modelon Impact can help you with your system simulation and product design journey.